6 Particles and temperature
Elements in various combinations comprise all matter, including living things. Some of the most abundant elements in living organisms include carbon, hydrogen, nitrogen, oxygen, sulfur, and phosphorus. These form the nucleic acids, proteins, carbohydrates, and lipids that are the fundamental components of living matter. Biologists must understand these important building blocks and the unique structures of the atoms that comprise molecules, allowing for cells, tissues, organ systems, and entire organisms to form.
All biological processes follow the laws of physics and chemistry, so in order to understand how biological systems work, it is important to understand the underlying physics and chemistry.
For example
The flow of blood within the circulatory system follows the laws of physics that regulate the modes of fluid flow. The breakdown of the large, complex molecules of food into smaller molecules—and the conversion of these to release energy to be stored in adenosine triphosphate (ATP)—is a series of chemical reactions that follow chemical laws. The properties of water and the formation of hydrogen bonds are key to understanding living processes. Recognising the properties of acids and bases is important, for example, to our understanding of the digestive process. Therefore, the fundamentals of physics and chemistry are important for gaining insight into biological processes.
At its most fundamental level, life is made up of matter. Matter is any substance that occupies space and has mass. Elements are unique forms of matter with specific chemical and physical properties that cannot break down into smaller substances by ordinary chemical reactions. There are 118 elements, but only 98 occur naturally. The remaining elements are unstable and require scientists to synthesise them in laboratories.
Each element is designated by its chemical symbol, which is a single capital letter or, when the first letter is already “taken” by another element, a combination of two letters. Some elements follow the English term for the element, such as C for carbon and Ca for calcium. Other elements’ chemical symbols derive from their Latin names. For example, the symbol for sodium is Na, referring to natrium, the Latin word for sodium.
The four elements common to all living organisms are oxygen (O), carbon (C), hydrogen (H), and nitrogen (N). In the non-living world, elements are found in different proportions, and some elements common to living organisms are relatively rare on the earth as a whole, as Table 2.2 shows. The atmosphere is rich in nitrogen and oxygen but contains little carbon and hydrogen, while the earth’s crust, although it contains oxygen and a small amount of hydrogen, has little nitrogen and carbon. In spite of their differences in abundance, all elements and the chemical reactions between them obey the same chemical and physical laws regardless of whether they are a part of the living or nonliving world.
Table 2.2 Approximate percentage of elements in living organisms (humans) compared to the non-living world
Element |
Life (Humans) |
Atmosphere |
Earth’s Crust |
Oxygen (0) |
65% |
21% |
46% |
Carbon (C) |
18% |
trace |
trace |
Hydrogen (H) |
10% |
trace |
0.1% |
Nitrogen (N) |
3% |
78% |
trace |
Atoms and molecules
All matter on Earth is made up of chemicals. Some materials, like rocks, can be simple mixtures or pure substances (for example, calcite, which is crystalline calcium carbonate). Living organisms are complex mixtures of thousands of chemicals. Everyday items are often fairly pure chemicals, with trace impurities that usually don’t affect their function or safety. A pure material, or homogeneous substance, consists of particles that behave chemically the same way. When we divide pure materials into smaller parts, we eventually reach a point where they no longer behave the same. The smallest unit that retains the chemical properties of the substance is called a molecule. In some cases, such as noble gases (helium, neon, argon) and pure metals, the smallest unit is an atom. Molecules can be broken down into atoms, which have different chemical behaviours. Atoms and molecules occupy space and have mass, making them matter. They can move freely unless compressed by external forces or bound together by attractive forces, as seen in solids and liquids.
For example
Example of an atom: Helium (He) is a noble gas consisting of single atoms
Example of a molecule: Water (H₂O) is a molecule made of two hydrogen atoms and one oxygen atom bonded together
Structure of an atom
To understand how elements come together, we must first discuss the element’s smallest component or building block, the atom. An atom is the smallest unit of matter that retains all of the element’s chemical properties. For example, one gold atom has all of the properties of gold, like its chemical reactivity. A gold coin is simply a very large number of gold atoms moulded into the shape of a coin and contains small amounts of other elements known as impurities. We cannot break down gold atoms into anything smaller while still retaining the properties of gold.
An atom is composed of two regions: the nucleus, which is in the atom’s centre and contains protons and neutrons. The atom’s outermost region holds its electrons in orbit around the nucleus (Figure 2.2). Atoms contain protons, electrons, and neutrons, among other subatomic particles. The most common isotope of hydrogen (H) is the only exception and is made of one proton and one electron with no neutrons.
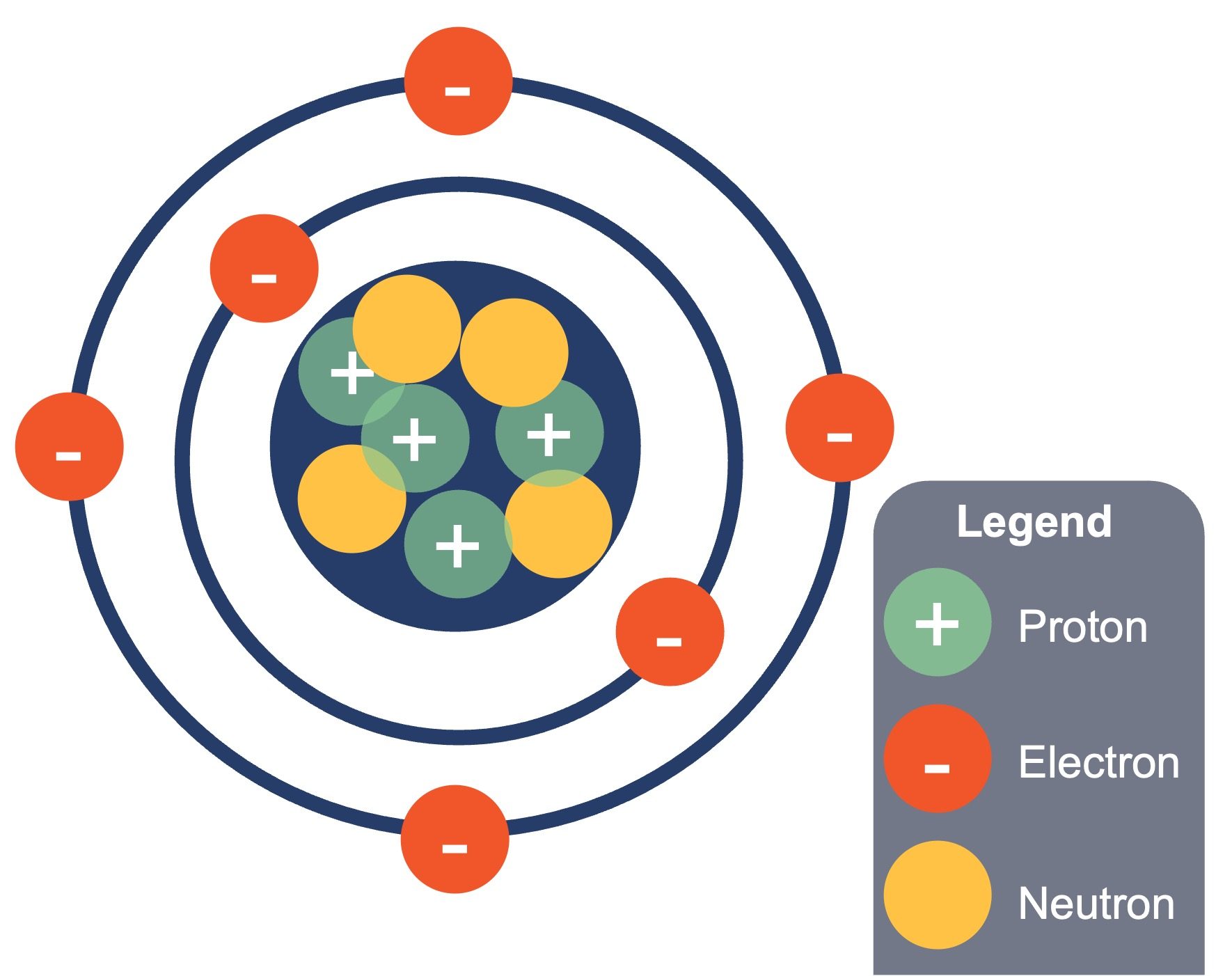
Atoms and potential energy
Atoms are the fundamental building blocks of matter, and they tend to combine with other atoms because doing so lowers their potential energy, making them more stable. When atoms combine, they form molecules. However, some atoms, such as those of noble gases like helium, neon, and argon, are very stable on their own and do not typically combine with other atoms. These noble gases exist as individual atoms under normal conditions. Similarly, noble metals like gold and platinum are often found in pure forms, such as gold nuggets, and do not readily combine with other elements.
In contrast, many common elements, such as hydrogen, oxygen, and nitrogen, are found throughout the universe and usually form molecules by joining with other atoms. For example, hydrogen gas (H₂) consists of two hydrogen atoms bonded together, while oxygen gas (O₂) and nitrogen gas (N₂) are made up of two oxygen and two nitrogen atoms, respectively. Chlorine gas (Cl₂) follows the same pattern. Additionally, oxygen can form ozone (O₃) when exposed to ultraviolet light from the sun.
All substances in the world are composed of either atoms or molecules. Molecules can consist of the same type of atom or different types. While noble gases are typically found as individual atoms, most other elements form molecules. These particles, whether they are pure substances like salt or mixtures like seawater or wood, make up everything around us.
Protons and neutrons have approximately the same mass, about 1.67 × 10-24 grams. Scientists arbitrarily define this amount of mass as one atomic mass unit (amu) or one Dalton (Table 2.3). Although similar in mass, protons and neutrons differ in their electric charge. A proton is positively charged; whereas, a neutron is uncharged. Therefore, the number of neutrons in an atom contributes significantly to its mass, but not to its charge. Electrons are much smaller in mass than protons, weighing only 9.11 × 10-28 grams, or about 1/1800 of an atomic mass unit. Hence, they do not contribute much to an element’s overall atomic mass. Therefore, when considering atomic mass, it is customary to ignore the mass of any electrons and calculate the atom’s mass based on the number of protons and neutrons alone. Although not significant contributors to mass, electrons do contribute greatly to the atom’s charge, as each electron has a negative charge equal to the proton’s positive charge. In uncharged, neutral atoms, the number of electrons orbiting the nucleus is equal to the number of protons inside the nucleus. In these atoms, the positive and negative charges cancel each other out, leading to an atom with no net charge.
Accounting for the sizes of protons, neutrons, and electrons, most of the atom’s volume—greater than 99 percent—is empty space. With all this empty space, one might ask why so-called solid objects do not just pass through one another. The reason they do not is that the electrons that surround all atoms are negatively charged and negative charges repel each other.
Table 2.3 Charge, mass and location of protons, neutrons and electrons
Charge |
Mass (amu) |
Location |
|||
Proton |
+1 |
1 |
nucleus |
||
Neutron |
0 |
1 |
nucleus |
||
Electron |
-1 |
orbitals |
Atomic number and mass
Atoms of each element contain a characteristic number of protons and electrons. The number of protons determines an element’s atomic number, which scientists use to distinguish one element from another. The number of neutrons is variable, resulting in isotopes, which are different forms of the same atom that vary only in the number of neutrons they possess. Together, the number of protons and neutrons determine an element’s mass number (Figure 2.3). Note that we disregard the small contribution of mass from electrons in calculating the mass number. We can use this approximation of mass to easily calculate how many neutrons an element has by simply subtracting the number of protons from the mass number. Since an element’s isotopes will have slightly different mass numbers, scientists also determine the atomic mass, which is the calculated mean of the mass number for its naturally occurring isotopes. Often, the resulting number contains a fraction. For example, the atomic mass of chlorine (Cl) is 35.45 because chlorine is composed of several isotopes, some (the majority) with atomic mass 35 (17 protons and 18 neutrons) and some with atomic mass 37 (17 protons and 20 neutrons).
Figure 2.3 Atomic number and mass (Charles Sturt University).
Reflective question
How many neutrons do carbon-12 and carbon 13 have, respectively?
Principal physical states of pure substances
Solids have a definite shape and volume (such as a rock or an ice cube). In solids, particles are tightly packed together in a fixed arrangement. They don’t move freely but vibrate in place. Imagine a group of people standing shoulder-to-shoulder, each person just slightly wiggling in their spot. This limited movement is why solids maintain their shape.
Liquids have a definite volume but take the shape of their container (such as water in a glass). Particles in liquids are close together but not in a fixed position. They can slide past each other, allowing the liquid to flow (we can imagine a crowd at a concert, where people can move around each other). This movement gives liquids their ability to flow and take the shape of their container.
Gases do not have a definite shape or volume. They expand to fill any container (such as air in a balloon). Particles in gases are far apart and move freely at high speeds (imagine a group of people in a large open space, running around in all directions without bumping often). This high-energy movement allows gases to expand and fill any available space.
If the number of particles in a substance remains constant across solid, liquid, and gas states (conservation of mass) in a container. The distribution of particles would vary (Figure 2.4). Solids particles are tightly packed and vibrate in place, held by strong forces, giving solids a fixed shape. Liquid particles are close together but move randomly, allowing them to flow and take the shape of their container’s bottom. Gas particles are far apart and move freely in straight lines, with high kinetic energy overcoming attractive forces, allowing them to fill the entire container.
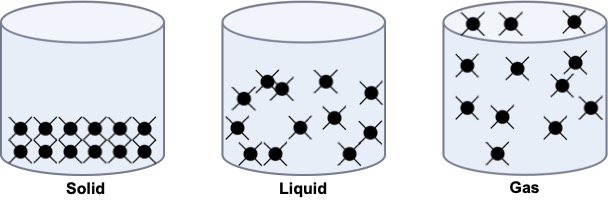
Temperature and particle movement
Understanding temperature and its effects on particle movement is crucial in science, as it influences various physical and chemical processes. Temperature measures the mean kinetic energy of particles. Kinetic energy, influenced by velocity and mass, is defined by the equation:
k = ½mv²
Temperature is proportional to the average energy with which particles collide and the maximum force they can impart during collisions. Absolute zero (0 K) is the point where particles have no kinetic energy and do not move. At this temperature, particles cannot diffuse or move along a gradient. Absolute zero is 0 K (Kelvin), while the Celsius scale is based on the freezing (0 °C) and boiling points (100°C) of water at standard atmospheric pressure (101325 Pa). Water freezes/ice melts at 0 °C (273.15 K) and boils at 100°C (373.124 K) under standard pressure. For reference, 273 K is the absolute temperature for 0 °C, and ~310 K is human body temperature. Mammals regulate their body temperature through homeostasis, maintaining a narrow range of core temperatures essential for optimal physiological function. This regulation involves mechanisms like sweating, shivering, and altering blood flow to the skin. Maintaining these narrow temperature ranges is crucial for enzyme function, metabolic processes, and overall health in mammals.
For example
Humans maintain a core temperature around 37°C (98.6°F). When our body temperature rises, sweating and increased blood flow to the skin help dissipate heat. Conversely, shivering and reduced blood flow to the skin help conserve heat when body temperature drops.
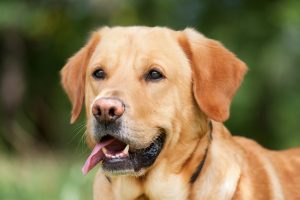
Dogs have a slightly higher core temperature, typically between 38.3°C and 39.2°C (101°F to 102.5°F). They regulate heat primarily through panting and limited sweating through their paw pads.
Horses maintain a core temperature around 37.5°C to 38.5°C (99.5°F to 101.3°F). They regulate temperature through sweating, which is more efficient than in many other mammals.
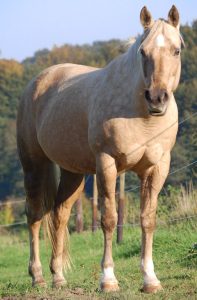
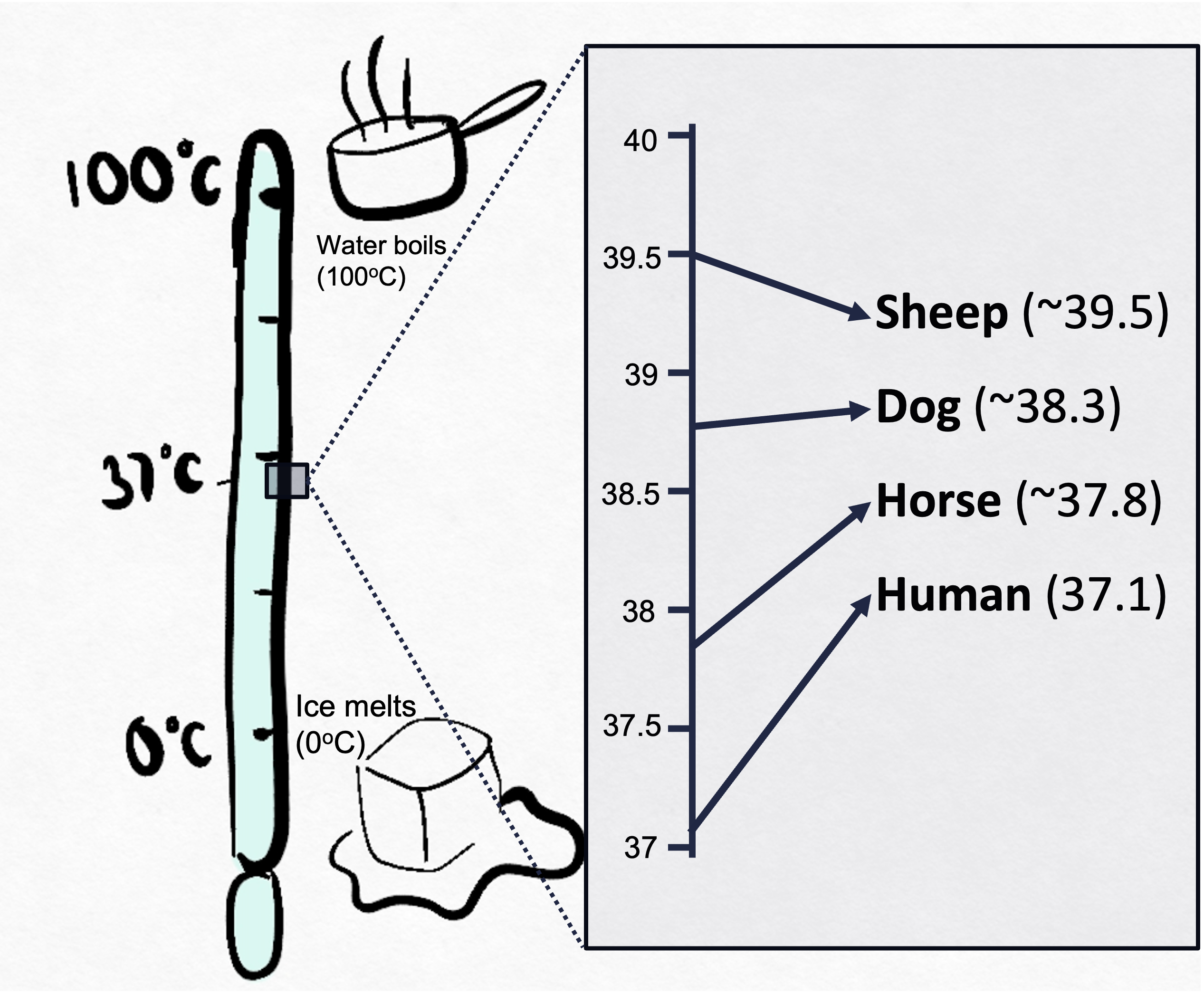
Particles behave differently under varying conditions, influenced by temperature and pressure (Figure 2.6). In solids, increased pressure doesn’t significantly alter particle arrangement. However, in liquids and gases, increased pressure compresses particles, making them more ordered and reducing their energy. Conversely, raising the temperature increases particle energy in liquids and gases, causing them to expand and occupy more space, and increasing vapour production.
For example
When administering anaesthetic gases to animals, understanding these principles is crucial. Increasing the pressure of the gas in the delivery system ensures it remains in a compressed, liquid state. As the gas is released and exposed to body temperature, it expands and vaporises, effectively delivering the anaesthetic to the animal. This helps veterinarians control dosage and ensure the safety of the animal during a procedure.
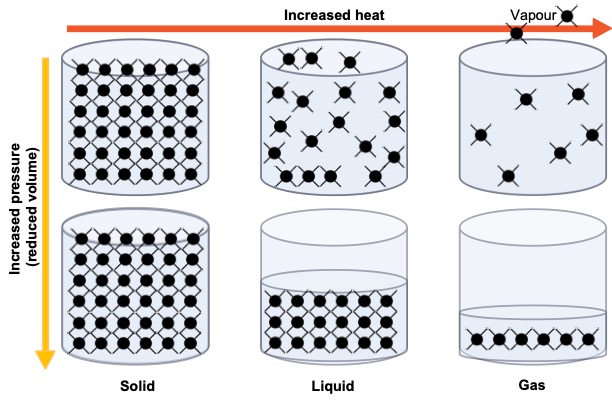
In a gas, particles occupy a defined volume, and their movement is influenced by temperature. This applies to all types of gases. An ‘ideal’ gas assumes that individual particles have no volume and do not interact with each other. When gas particles collide with the walls of a container, they exert force, creating pressure (P). Many particles colliding randomly over time produce an even force across the surface. If we double the number of particles (n) in the container, collisions with the walls double, thus doubling the pressure. Therefore, (n) is proportional to (P). Doubling the absolute temperature (T) doubles the energy per collision, also doubling the pressure. Therefore, (T) is proportional to (P). Compressing the container to half its volume (V) doubles the number of collisions per unit space, doubling the pressure. Hence, (V) is inversely proportional to (P).
These relationships are described by the Universal Gas Law:
PV = nRT
Where R is the universal gas constant, equal to 8.31446261815324 when using base SI units (with pressure in kPa).
At Standard Temperature and Pressure (STP), which is 273.15 K and 1 atmosphere (101.325 kPa), one mole of any gas occupies 22.414 litres. This value is for an ideal gas, which is a theoretical concept.
Reflective question
What are the underlying reasons that gases in a container exert pressure, and how can this pressure be measured?
(Hint: Consider how particle collisions can modify the pressure within the container)