26 Lipids
What are lipids?
Lipids (also known as fats) are components of plant (for example, vegetable oils) and animal tissues (for example, meat, eggs, milk). On a physical nature, lipids are relatively insoluble in water and are soluble in organic solvents, such as hexane, ether, and chloroform.
Lipids (fats) are important in the nutrition of food-producing animals. After carbohydrates, lipids serve as a major source of energy in animal diets.
Chemically, lipids are organic compounds and esters of fatty acids and glycerol (a 3 C compound) or some other alcohol. Fats are the primary storage form of energy (for example, oil in seed). For example, the abdominal fat pads in chicken and back fat in pigs are mostly triglycerides.
Classification of lipids
1. Simple lipids:
- Commonly called triglycerides (or triacylglycerol) are esters of fatty acid with alcohol, e.g. 1 glycerol + 3 fatty acids.
2. Compound:
- Steroids
- Phospholipids
- Eicosanoids
3. Derived Lipids
Simple lipids like triglycerides are more common and are an important component in animal rations (e.g., vegetable oil and animal fats such as tallow or lard). Compound lipids are composed of a lipid plus a non-lipid molecule (e.g., protein). Lipoprotein (lipid + protein) are examples of compound lipids and are used for lipid transport (like a courier). Within the animal body, compound lipids are more important in physiology and metabolism (e.g., lipid transport, phospholipids as part of cell membranes). As implied by their name, derived lipids originate from simple or compound lipids through hydrolytic processes. Examples of derived lipids include sterols, fatty acids, and fat-soluble vitamins.
Why add fats to animal diets?
Nutritionally, fats are excellent sources of energy and are essential to the survival of animals. Fats are the sole source of essential fatty acids (those that cannot be made by the body) for animals. Fats can also provide fat-soluble vitamins. However, this role is very minimal in livestock as feeds are supplemented with vitamins.
The most important role of dietary fat is to provide essential fatty acids.
As the fat content of the diet increases, the energy density of the diet goes up.
Physically, the addition of fats is associated with the improvement of feed quality, the reduction of dust in feed, the reduction of feed particle separation during processing, an increase in palatability, an increase in digestive lubrication (i.e., emulsification and rate of passage), and an increase in feed digestibility.
What are fatty acids?
Fatty acids are the main players in lipid nutrition. This is due to their diversity in structure, composition, and ability to be metabolised. The molecular composition of a fatty acid includes a hydrophilic carboxyl group (−COOH) and a hydrophobic methyl group (−CH3) at opposite terminals of a hydrocarbon backbone (Figure 5.12).
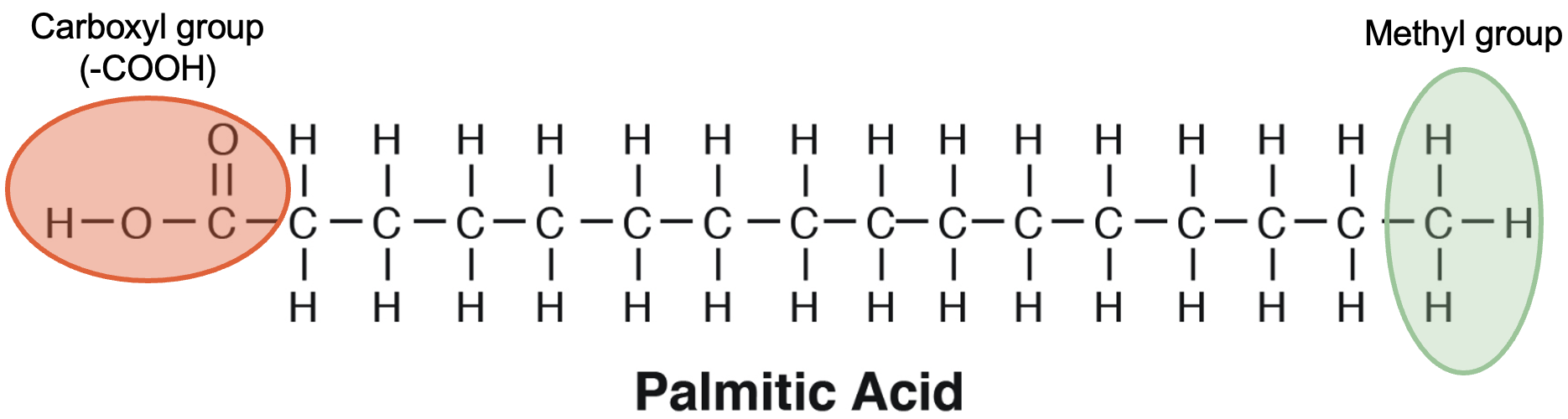
In most cases, three fatty acids are attached to the glycerol molecule and are called triacylglycerol. The three fatty acids in triacylglycerol can differ in chain length (i.e., total carbons in the fatty acid molecule) as well as in the number of double bonds.
Fatty acid composition and structure determine the physical property and nutritional quality of fats. For example, when there is a predominance of saturated fats in the triacylglycerol, fat tends to solidify (e.g., fat around a piece of meat), and when there is a predominance of unsaturated fats, fat tends to liquefy (e.g., salad oil).
Physical properties of fatty acids
- An increase in saturation makes fats more solid.
- An increase in unsaturation makes fats more liquid or decreases their melting point.
Fatty acids are classified into three families based on the presence (or absence) of double bonds in the hydrocarbon chain. These include saturated fatty acids, monounsaturated fatty acids, and polyunsaturated fatty acids (PUFAs).
Acids
- Saturated = no double bonds
- Unsaturated = presence of double bonds (could be one or two)
- Polyunsaturated = more than two double bonds
Saturated fatty acids are “saturated” with hydrogen or straight chains with no double bonds (e.g., palmitic acid, C16:0). When there is a predominance of saturated fats in the glycerol moiety, the triacylglycerol tends to be solid. This is because due to their straight chain nature, they tend to “pack” very tightly in the membrane (e.g., tallow or beef fat; Figure 5.13).

Unsaturated fatty acids contain one or more double bonds between adjacent carbon atoms in the hydrocarbon chain. Unsaturated fatty acids may be either mono (one double bond) (Figure 5.14) or polyunsaturated (more than two double bonds) (Figure 5.15). When there is a predominance of unsaturated fats, the triglyceride tends to be liquid because unsaturation gives a “bend” in their structure and they cannot pack as tightly as saturated fats (e.g., vegetable oil) .

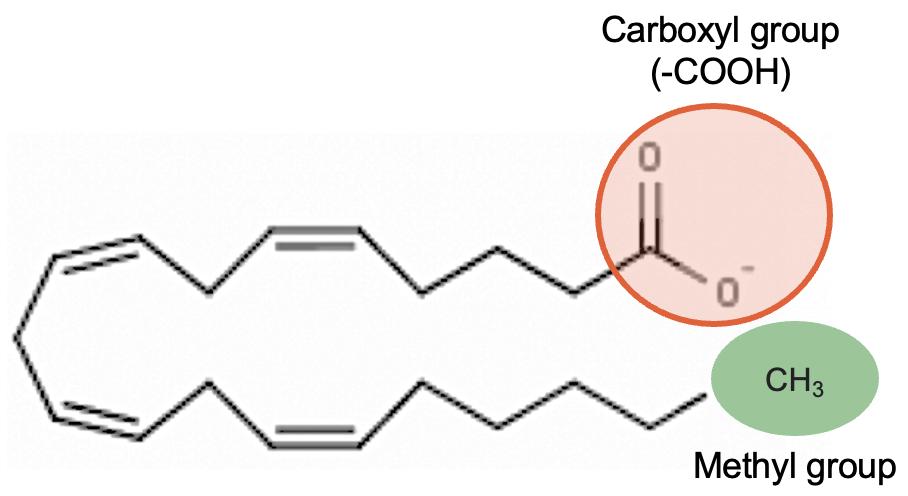
Polyunsaturated fatty acids are commonly called “PUFA” and contain two or more double bonds. Due to these extra double bonds, PUFAs tend to be more “round” when compared to the straight chain structure of a saturated fat. These double bonds also change the physical nature of the fat, making it more liquid than straight chain saturated fat. In addition to the number of double bonds, the position of double bonds in the carbon-carbon chain is also important in nutrition and in the metabolism of lipids; this is explained below.
Nutritionists designate the term omega (ω) or “n” to denote the position of double bonds in the carbon chain in a PUFA. The omega carbon is the first carbon with a double bond counting from the methyl end (CH3) of the carbon chain. The two PUFA classifications are omega-6 (also called n-6, or ω-6) or omega-3 (n-3, or ω-3). For instance, omega-3 fatty acid will have the first double bond at the third carbon when counted from the methyl (CH3) end (Figure 5.16a) and omega-6 fatty acids will have the first double bond at the sixth carbon when counted from the methyl (CH3) end (Figure 5.16b). The locations of the double bonds are also indicated by the Greek letter Δ, “delta,” in some chemistry or biochemistry textbooks. The delta term denotes the position of double bonds from the carboxyl end. However, the term omega, or “n,” is the one that is commonly used by nutritionists.
Two types of PUFA:
- Omega-6 (n-6, or ω-6) fatty acid
- Omega-3 (n-3, or ω-3) fatty acid
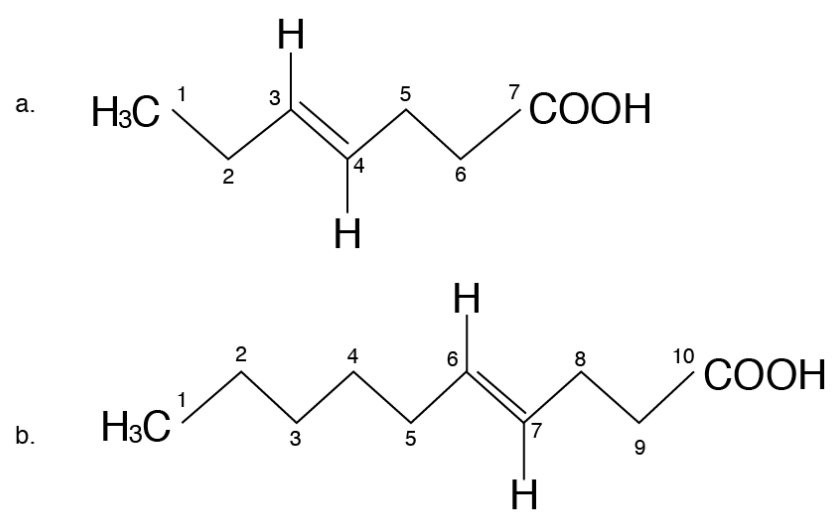
Essential fatty acids
In nonruminant, or monogastric, animals such as pigs, two fatty acids (α-linolenic acid, C18:3 n-3) and linoleic acid (C18:2 n-6) have to be supplied in the diet and are called essential fatty acids. This essentiality is due to the inability to insert double bonds at the third and sixth carbon from CH3 end in n-3 and n-6 locations. In addition to these two essential fatty acids, carnivores such as cats need arachidonic acid (C20:4 n-6) in their diets. In nutrition, the term “essential” means animals cannot synthesise it to meet their requirements.
Example
Essential fatty acids include:
- Linoleic acid (C18:2 n-6)
- Linolenic acid (C18:3 n-3)
- Arachidonic acid (C20:4 n-6; in true carnivores, e.g., cats)
Fatty acid nomenclature
Fatty acids are commonly expressed by their trivial names (e.g., linoleic acid) or their associated shorthand notations (C18:2 n-6). The shorthand nomenclature of a fatty acid includes the number of carbon atoms and double bonds. For instance, in linolenic acid, C18:2 n-6 stands for 18 carbon atoms and two double bonds, of which the first double bond is at the sixth carbon atom from the methyl carbon.
Cis and trans fatty acids
Unsaturated fatty acids can form geometric isomers, with either cis or trans, depending on the stereo-conformation of groups around a double bond. Most natural fatty acids of animal and plant origin are of the cis type, whereas those of bacterial origin contain both cis and trans types.
Example
Conjugated linoleic acid (CLA) is a trans fatty acid present in cow’s milk or other ruminant food like beef and is produced by rumen microbes during the biohydrogenation process. In CLA, the two double bonds lack a methylene group separating them, have a conjugated arrangement, and are called natural trans fats. Trans fats such as CLA have received considerable attention due to their several health-promoting (e.g., anticancer, immune health enhancing, lean body mass enhancing) effects. There are other trans fats that are produced during the hydrogenation process (the addition of hydrogen) when liquid vegetable oil is made into solid fats such as margarine. These are synthetic trans fats and have different health effects when compared with “natural” trans fats such as CLA.
Cis versus Trans Fatty Acids
- Most natural fats occur in the cis form.
- The exception is a trans fat called conjugated linoleic acid (CLA; C18:2 n-6), which is produced by rumen microbes.
Cholesterol
Sterols (lipids with phenanthrene ring–like structures) are the most abundant steroid in the human diet. Cholesterol is the best known steroid (fat-soluble substance containing a steroid nucleus) and is the precursor of many other substances such as vitamin D, bile acids, sex hormones, and corticosteroid hormones.
An important component of animal tissues, egg yolks, and cell membranes, cholesterol synthesis is partly by dietary intake and partly by biosynthesis from acetyl CoA. Excess cholesterol is stored in arteries and can lead to atherosclerotic plaque formation and cardiovascular disorders. Excretion of cholesterol is through bile acid formation. Plant cells do not contain cholesterol but instead contain other sterols called phytosterols.
Monogastric animals
The digestion process involves the breakdown of lipid molecules into smaller ones that are eventually absorbed into the blood. Lipids are not soluble in water, which is the aqueous medium of the digestive tract (lipids are hydrophobic). Therefore, the initial step in lipid digestion is to make them dissolve in water. How? Through a process called emulsification, or the dispersion of lipids in small droplets.
Emulsification is the dispersion of lipids in small droplets.
Dietary lipids (mostly triglycerides), upon their entry into the small intestine, are emulsified by bile salt (also called bile acid) released from the gall bladder. Bile salt functions as a detergent (due to their OH and COOH groups), and large lipid molecules form smaller lipid droplets surrounded by a layer of bile. Emulsified lipids are acted upon by enzyme pancreatic lipase and converted into fatty acids, monoglycerides and glycerol.
The lipid digestion products are assembled into micelles. These are temporary combinations of bile salt, fatty acids, monoglycerides, and other fat-soluble substances such as vitamins and cholesterol. The micelles are water soluble and enable the lipid digestion products to be transported to the small intestinal surface for absorption. At the site of absorption, the micelle breaks down and the bile salt returns to the intestine for continuing emulsification processes (bile salt recycling). The components are absorbed into the small intestine by passive diffusion. In a nutshell, the ability to form micelles and the presence of bile salt are very important for lipid digestion, and the lack of it can affect digestibility. For example, saturated fatty acids are less efficient than unsaturated fatty acids in forming micelles. So a blend of saturated and unsaturated fatty acids is used in animal rations.
Micelles and chylomicrons are temporary compounds formed during lipid absorption.
Once inside the intestinal cell (or enterocyte), the monoglycerides and fatty acids are reesterified, and together with free and esterified cholesterol, lipoproteins and phospholipids are assembled into chylomicrons. The chylomicrons are secreted into the lymphatic system.
Ruminant animals
In ruminant animals, the lipid content of the diet is low (under 5%) and comes from different sources such as grass, leaves, oil seeds, or cereal grains. Leaf or grass lipids are mainly galactolipids, phospholipids, waxes, pigments, and essential oils, and oil seed or grain lipids are mainly triglycerides.
In the rumen, there is no emulsifying agent or pancreatic lipase enzyme. Instead, there are rumen microbes producing microbial lipases. When dietary lipids enter the rumen, the initial step is the hydrolysis of the ester linkages in triglycerides, phospholipids, and glycolipids. Hydrolysis of dietary lipids is done by microbial lipases, which releases glycerol and fatty acids (free fatty acids) from the lipid backbone. Glycerol is readily metabolised by the rumen bacteria to form propionic acid. Feeding of supplemental fat increases the proportion of propionic acid (one of the volatile fatty acids, or VFAs) and the propionate:acetate ratio in ruminants. Hydrolysis is a prerequisite for the next step.
Lipids undergo hydrolysis, biohydrogenation, and conjugated fatty acid formation in the rumen.
Biohydrogenation of unsaturated fatty acids is the second major transformation that dietary lipids can undergo in the rumen. Fatty acids with double bonds are altered by microbes to form more stable fatty acids. Fatty acids such as linoleic acid are converted “conjugated” fatty acids (e.g., conjugated linoleic acid, or CLA) in which the double bonds are not separated by methylene (CH2) groups. The position of double bonds is altered, and the fatty acids are converted to more stable “trans” fats. Some odd-numbered (e.g., C19:0) and branched-chain fatty acids are also created during this process. For example, linoleic acid (C18:2 n-6), where the double bonds are in the cis position (cis9-cis12), is converted to several isomers of CLAs during this conversion step.
Reflective question
Why biohydrogenation?
Too much unsaturated fatty acids can be toxic to rumen microbes.
Lipid digestion in the ruminant small intestine is very similar to lipid digestion in monogastric animals. The two key secretions enabling this process are bile and pancreatic juices. These secretions enable the lipids to form micelles for absorption. Bile supplies bile salts and pancreatic juice and enzymes. These compounds desorb the fatty acids from feed particles and bacteria, allowing the formation of micelles. Once micelles are formed, they facilitate the transfer of water-insoluble lipids across the intestinal epithelial cells of the jejunum, where the fatty acids are absorbed. Within the intestinal epithelial cells, the fatty acids are reesterified into triglycerides and then packaged into chylomicrons for transport in lymph to the blood.
Lipid Transport: Blood lipids consist of chylomicrons formed within the intestinal mucosal cells during absorption as well as lipids derived from storage depots, such as liver and adipose tissue. Blood lipids are transported as lipoproteins due to their hydrophobic nature.
Lipids are transported as lipoproteins in the blood: Lipoproteins consists of an inner core of hydrophobic lipids surrounded by a surface layer of phospholipids, cholesterol, and outer proteins (apolipoprotein).
Main lipoproteins
- Chylomicron
- VLDL
- LDL
- HDL
Lipoproteins are a lipid + a protein (compound lipid). Because lipids are less dense than water, the density of lipoproteins decreases as the proportion of lipid to protein increases. Lipoproteins have a major role in lipid and cholesterol transport and metabolism.
Lipoproteins are classified based on their density and composition. The main lipoproteins in blood are chylomicrons, very-low-density lipoproteins (VLDL), low-density lipoproteins (LDL), and high-density lipoproteins (HDL).
Chylomicrons are synthesised in the small intestine from dietary fat, and VLDL, LDL, and HDL are synthesised in the liver and small intestine. Chylomicrons enter the liver and are packaged into VLDL. VLDL is involved in the transport of triacylglycerol (TAG) from the liver to extrahepatic tissues. LDL transports cholesterol (so-called bad cholesterol) to tissues and HDL is responsible for “reverse transport” or removal of cholesterol (so-called good cholesterol) from tissues. The terms “bad” and “good” refer to the nature of transport. For example, the “good” cholesterol refers to cholesterol being carried by HDL, which is meant to be routed to the liver for bile formation, or excretion, thus leaving the body and not deposited, like LDL, into blood vessels.
Fatty acid metabolism
The liver has a central role in lipid transport and metabolism as it is involved in the synthesis and catabolism of lipoproteins. Pathways of fatty acid degradation and biosynthesis are highly intertwined with pathways of carbohydrate metabolism due to the central role of acetyl CoA in both lipid and carbohydrate metabolism.
Fatty acid oxidation
Fatty acids released from the hydrolysis of TAG are transported via blood. Oxidation occurs in the mitochondria of tissues, such as skeletal muscle, cardiac tissue, liver tissue, adipose tissue, and other tissues by converting them to acetyl CoA, which is then catabolised in the citric acid cycle.
Fatty acids are oxidised in the mitochondria by converting them to acetyl CoA.
Before oxidation begins, fatty acids are activated (they react with CoA) to form fatty acyl CoA (ATP is needed for this step). Activated fatty acids are transported across the mitochondrial membrane (carnitine serves as a carrier). In β-oxidation, two carbons at a time are cleaved off from fatty acid CoA, starting from the carboxyl end. The hydrogen produced from fatty acids are taken up the chain by hydrogen acceptors (e.g., FAD, NAD+) and produces a high yield of ATP. Acetyl CoA released can enter the tricarboxylic acid (TCA) cycle for ATP production, can form ketone bodies, or can be used for resynthesis of fatty acids.
For example, during the β-oxidation of palmitic acid (C16:0), through degradation of two carbon fragments, total ATP and net ATP generated is shown below.
During the β-oxidation of palmitic acid (C16:0), through degradation of two carbon fragments, total ATP and net ATP generated is:
Carbon-carbon cleavage
7 cleavage (shown by red lines) = 5 ATP per each cleavage (1 FADH + 1 NADH)
Oxidation of acetyl CoA
8 acetyl CoA units entering the TCA cycle = 12 ATP per acetyl CoA unit
Total ATP for 1 mole of palmitic acid (C16:0) = 35 ATP + 96 ATP = 131 ATP
= 7 cleavage points (7 × 5 = 35 ATP) + 8 acetyl CoA units (8 × 12 = 96 ATP)
Net ATP generated by 1 mole of palmitic acid (C16:0) = 35 + 96 − 2 = 129 ATP
(Two ATP used for initial activation and are then subtracted from 131 ATP)
Fatty acids produce higher levels of ATP than carbohydrates because they are less oxidised.
Fatty acids of 16 C and 18 C are oxidised through the β-oxidation pathway. Other longer-chain fatty acids are oxidised in the peroxisomes. β-oxidation of unsaturated fatty acids occurs until the first double bond is reached. The double bonds are isomerised from cis to trans and then hydrolysed by isomerase, ultimately yielding acetyl CoA.
Lipogenesis and Fatty Acid Synthesis
Lipogenesis is the process of synthesising lipids as a means of storing chemical energy. Fat cells, or adipocytes, are dispersed throughout the body and are considered a long-term energy depot. Lipogenesis encompasses fatty acid synthesis (cytosol of hepatocytes and adipocytes), adipocyte uptake, and storage of lipids as the body’s “savings account.”
What Causes Fatty Acid Synthesis? Glucose is the key signal for fat storage (feasting). Excess energy (ATP) generated from glycolysis and the TCA cycle is taken up by the body to convert it to fat. As ATP levels increase beyond the cells’ requirements, the ATP begins to accumulate, which stimulates activity of the enzyme acetyl CoA carboxylase. Increased insulin concentrations are also required to stimulate acetyl CoA carboxylase activity.
Fatty acid biosynthesis begins with 2 C acetyl CoA (like β-oxidation). Acetyl CoA could come from fats, carbohydrates, or some amino acids. Fatty acid synthesis occurs in the cytosol (endoplasmic reticulum). The fatty acid chain is assembled in 2 C units (derived from acetyl CoA) by joining the carboxyl end of one fragment to the methyl tail of another, yielding palmitic acid (C16:0) as the end product.
Fatty acid synthesis occurs in the cytosol.
Briefly, acetyl CoA carboxylase uses one ATP to combine one mole of acetyl CoA to form malonyl CoA. Fatty acid synthesis begins with the addition of carbon dioxide to 2 C acetyl CoA to form 3 C malonyl CoA. Acetyl CoA carboxylase is the enzyme needed for this step. Malonyl CoA reacts with acetyl CoA to produce a 5 C intermediate compound, which is decarboxylated to form a 4 C butyryl CoA. This in turn then combines with another malonyl CoA and is decarboxylated to form 6 C caproyl CoA . The step is continued by adding malonyl CoA and losing CO2 each time to produce 16 C palmitic acid as the eventual final product.
Most fatty acids are even numbered because each acetyl CoA, and subsequent malonyl CoA, is only two carbons in length. Each malonyl CoA adds two more carbons, and the resulting fatty acid is almost always evenly chained. Some very minor odd-numbered fatty acids (e.g., C17:0, C19:0) are present in animal tissues.
Fatty acid synthase is the enzyme involved in these steps. Palmitic acid (16 C) can be elongated to form 18 C stearic acid by elongases. Further formation of unsaturated fatty acid (oleic acid) C18:1 is by the introduction of double bonds by the desaturase enzyme. Mammals cannot insert double bonds beyond ∆-9 position. Therefore, linoleic (18:2 ∆-9,12) and linolenic (18:3 ∆-9,12,15) need to be provided in the diet and are called essential fatty acids. However, upon consumption, mammals can further desaturate and elongate the 18 C essential fatty acids to form longer chain 20 and 22 C fatty acids.
Storage and Mobilisation of Lipids
Lipids are stored as TAG. The glycerol backbone of TAG is formed from dihydroxyacetone phosphate, produced from the preparatory phase of glycolysis (along with glyceraldehyde-3-phosphate). Glycerol is three carbons, and therefore, each carbon can bond together with a fatty acid. The bonding of the glycerol and fatty acids occurs mainly within the cytosol of hepatocytes or adipose tissue in ruminants (or the mammary gland in lactating animals). Once the TAGs have been formed, they are attached to lipoproteins and deposited in the blood for transport. The lipoprotein complexes are recognised by lipoprotein lipase, and the TAGs are removed and deposited as fat (e.g., fat pads in chickens, back fat in pigs).
Mobilisation of lipids from storage sources for energy production is through the action of hormone-sensitive lipoprotein lipase, which releases free fatty acids and glycerol. Mobilisation occurs during starvation (fasting), stress or increases in energy usage (e.g., disease). Hormones such as glucagon and epinephrine increase, and insulin is reduced under these conditions and stimulates the action of lipases (hormone sensitive).
In dairy cattle and in ewes during certain conditions such as negative energy balance, fat mobilisation is at its peak and may exceed the rate at which acetyl CoA enters the TCA cycle. During such conditions, hepatic synthesis of ketone bodies (ketogenesis) occurs. The ketone bodies are acetoacetic acid, β-hydroxybutyric acid, and acetone. Ketosis is a metabolic disorder when excessive quantities of ketone bodies are produced.
Example
Ruminant animals derive acetyl CoA directly from absorbed acetate (volatile fatty acid) rather than glucose. Citrate is permeable and is transported across the mitochondrial membrane and is converted to α-ketoglutarate with the production of nicotinamide adenine dinucleotide phosphate (NADPH). The α-ketoglutarate reenters mitochondria, while NADPH is used for fatty acid synthesis from acetate. It is a means for ruminants to conserve glucose and glucogenic precursors
Dive deeper
Watch this video on lipids: RicochetScience. (2016, April 13). Lipids [YouTube, 6:28mins]
Knowledge check