38 DNA and chromosomes
On this page
Every sexually reproducing organism begins life as a fertilised egg (embryo) or zygote.
All multicellular organisms use cell division for growth and the maintenance and repair of cells and tissues.
Chromosomes
The eukaryotic genome is made up of chromosomes. In diploid organisms, there are two copies of each chromosome. Comparatively, haploid organisms only have one copy of each chromosome.
For example
Humans have 23 pairs of chromosomes. These ‘pairs’ of chromosomes are similar in that they have all of the same genes in the same order, but they often carry different versions of the genes, known as alleles. One set of 23 chromosomes comes from our egg-bearing biological parent, and the other from our sperm-bearing biological parent.
During most of the cell’s life, each of these chromosomes will be made of a single chromatid, and that chromatid will exist as chromatin. Chromatin is a complex of DNA and proteins that help keep the DNA organised inside the nucleus.
If the cell plans to undergo meiosis or mitosis, then the DNA will be replicated so that each chromosome now is composed of two identical sister chromatids, which are exact copies of each other and are connected to each other via the centromere. In interphase, chromatin is in its more relaxed form, which allows access to the DNA. Despite this, not all of the DNA is equally relaxed: chromatin that is less condensed, allowing the genes in that area to be expressed, is called euchromatin. In contrast, heterochromatin is a form of chromatin that is less active and somewhat more compact. Just prior to mitosis or meiosis, all nuclear function is shut down, and the chromatin takes on its most condensed conformation to form the characteristic mitotic chromosomes.
Dive deeper
Watch this video that covers key terminology of the cell cycle: MITx Bio. (2015, August 27). Cell cycle terms [YouTube, 6:24mins]
DNA is very small. The diameter of the DNA double helix is roughly 2 nm. On the other hand, if you were to take all of the DNA in a human cell and line it up end to end, it would be over 2 metres long! The average nucleus of a human cell is 6 µm in diameter. So, then how does the cell pack all of that DNA in? The DNA must also be extremely organised so that each gene can be accessed quickly and accurately.
Chromatin is formed from DNA, histones and non-histone chromatin-associated proteins.
Chromatin is formed soon after replication, when the DNA is carefully folded and organised, with the help of proteins. However, when gene expression is required, specific regions of the packed DNA will be loosened (by shifting or removing some of the packing proteins) so that transcription factors, RNA polymerase, and other expression machinery can bind to the DNA and transcribe it.
Chromatin formation as levels of packing of the DNA:
- The initial association of the DNA with histones to form the ‘beads-on-a-string‘ structure
- The nucleosomes pull together to form a more tightly wound form of chromatin, called the chromatin fibre or 30 nm fibre
- Higher-order packing to form the most condensed forms, used for mitosis and meiosis
Histones
Histones are a set of proteins that interact strongly, but reversibly, with DNA. They are found in all eukaryotes, and even Archaea, but not bacteria. Histones are highly conserved proteins in all eukaryotes. They are considered to be ‘basic’ proteins due to the overall positive charge (due to high pKa) they carry in their amino acid sequence. This overall positive charge attracts the DNA due to the negative charge that is carried on the DNA backbone. There are five major types of histones that are used to help pack the DNA and produce chromatin:
- Core histones (H2A, H2B, H3, and H4) interact strongly with each other to form a core complex. DNA wraps around the outside of this core protein complex.
- Histone H1 binds to the outside of the nucleosome and helps pack the nucleosomes together to tightly pack the DNA.
The core histones come together in specific pairs to form a larger complex called an octamer (Figure 8.1). Most organisms have several different genes to represent each of the histone variants, which influences how tightly packed the chromatin is. This has a significant impact on how and when specific genes are expressed.
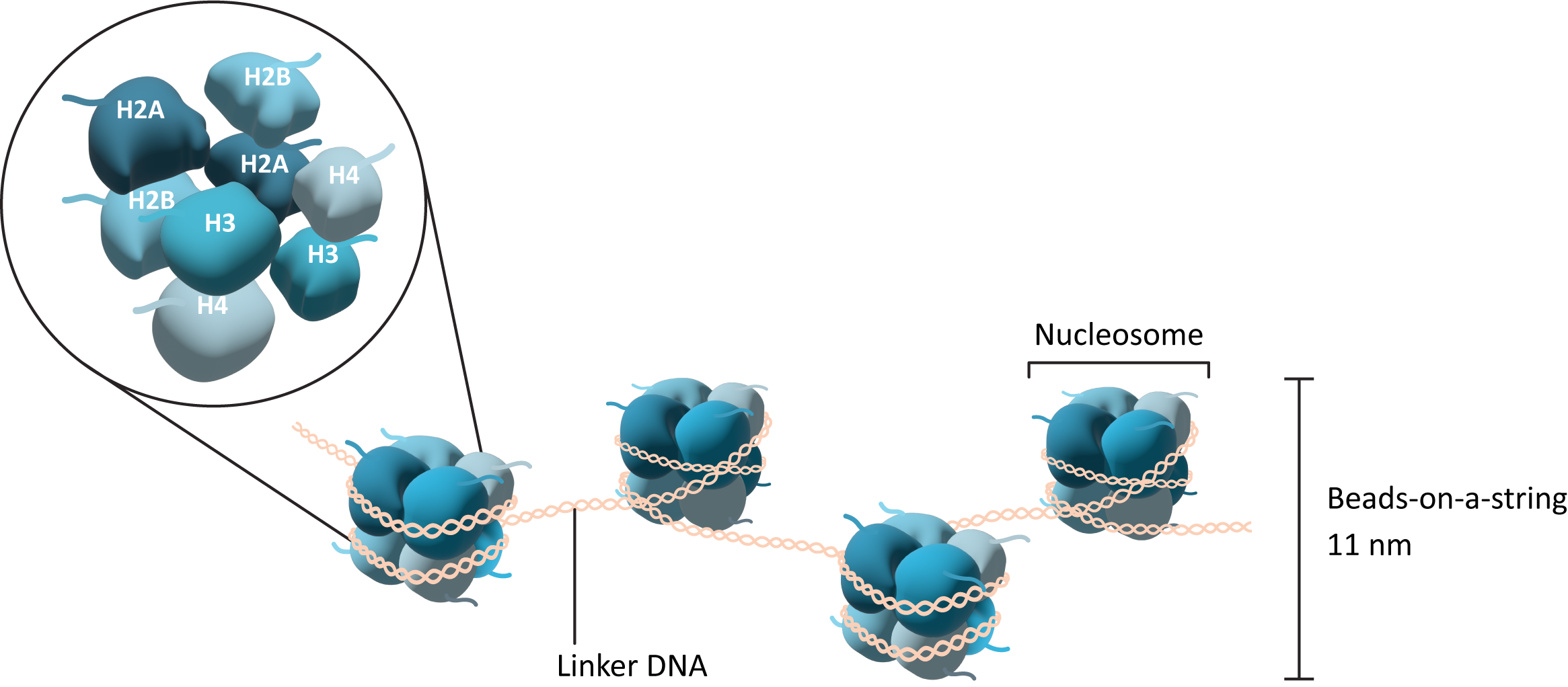
In addition to the histone proteins in the core, a fifth histone family exists known as H1. The H1 histone does not form part of the nucleosome core, but rather it sits on the surface of the nucleosome, on top of the DNA, and helps keep it in place. It also helps pull in the linker DNA so that the chromatin is more tightly packed (Figure 8.2).
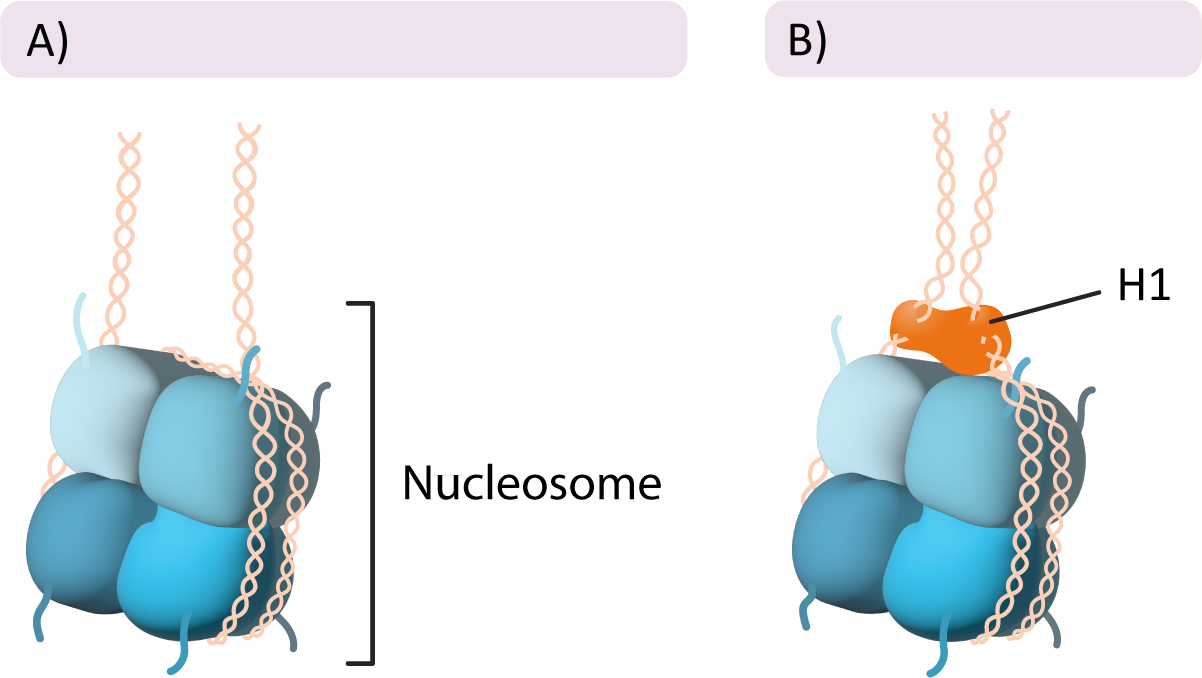
Organisation of the nuclear genome
There is a lot going on in the interphase nucleus. Some genes are being actively expressed, while others are being actively repressed and put away. Some parts of the genome don’t carry genes at all but instead are important structural regions that are needed to protect the DNA and help with mitosis. All of this is housed in an extremely tiny cellular compartment, the nucleus. Therefore, it is absolutely crucial that the contents of the nucleus remain as organised as possible at all times.
There are a couple of ways the cell manages to maximise space in the nucleus, the first of which is to ensure that only the DNA that is currently needed is unpacked enough to allow for gene expression, and everything else is tightly packed away. This leads to differences in the packing of interphase chromatin in different regions of the nucleus. The differences in packaging of chromatin euchromatin and heterochromatin changes how the chromatin looks in electron microscopy (Figure 8.3).
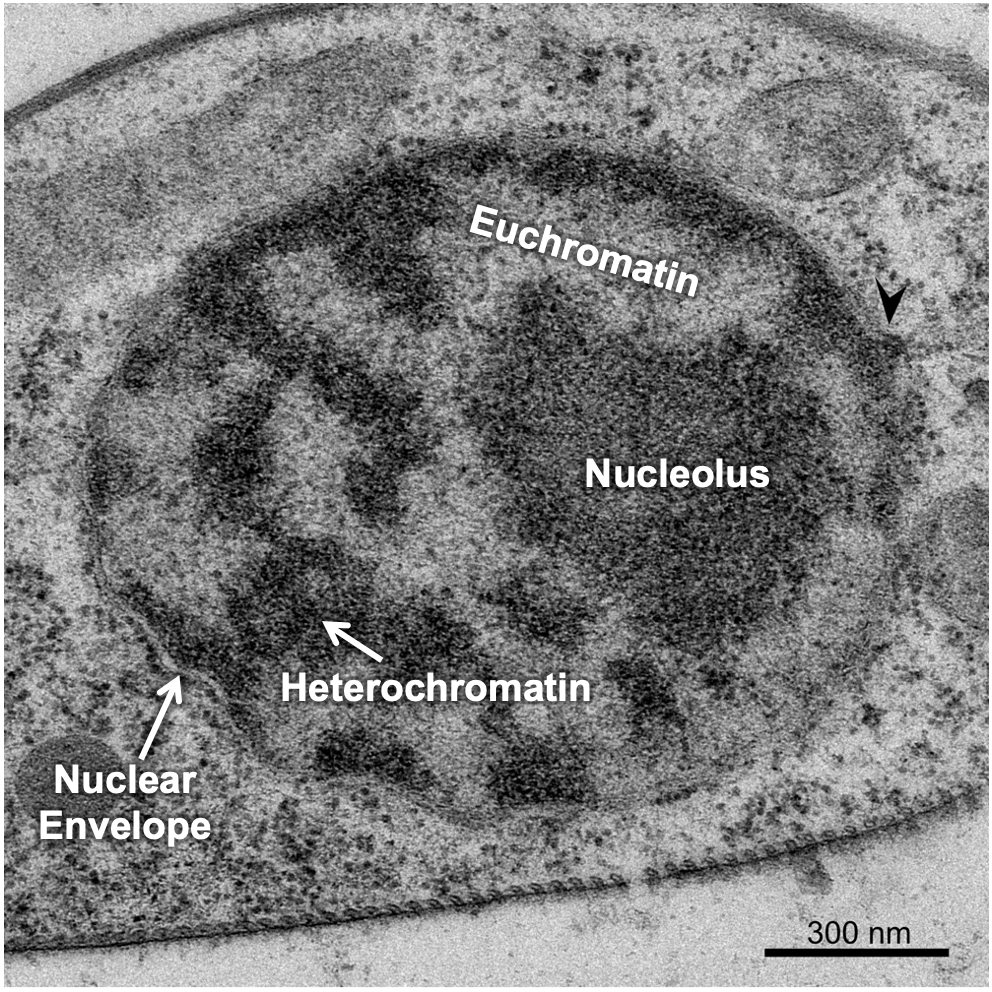
Reflective question
Why is the heterochromatin darkly stained and the euchromatin lightly stained in TEM?
Heterochromatin is more tightly packed form that has the H1 histone bound to it so that the nucleosomes form a spiral and pack together tightly (Figure 8.4). Heterochromatin does not allow proteins like transcription factors or polymerases to access the DNA. As a result, in these regions no gene expression can take place. At any given moment, most of the chromatin in a cell is in the form of heterochromatin. Euchromatin is the less-condensed form where DNA can be accessed. Active transcription is very likely taking place in these regions as well as other forms of gene regulation.
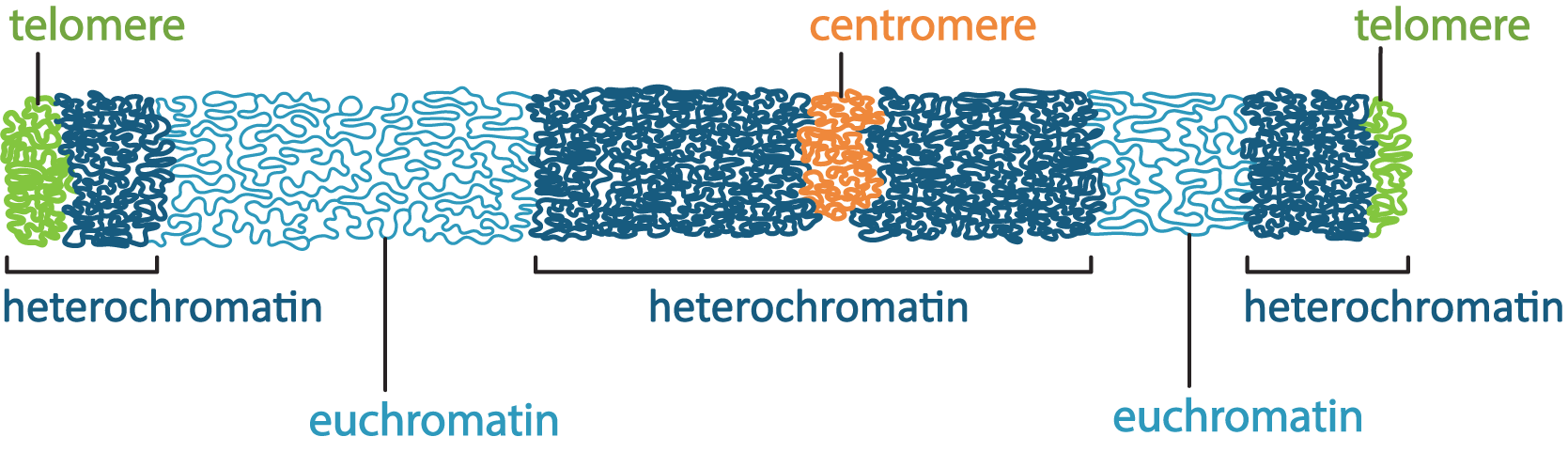
In any given cell, at any given moment, it will be expressing a specific subset of genes. The subset of genes being expressed may or may not be the same as a different cell. This is especially true of cells in different tissue types. A cell of the pancreas synthesising and secreting digestive enzymes will be expressing a very different set of genes than a neuronal cell, for example. In addition, cells will change the genes that they need to express over time. There are a number of genes that are only turned on during embryonic development and then get turned off.
Dive deeper
Watch this video to learn about DNA replication: yourgenome. (2015, June 26) DNA replication – 3D [YouTube, 3:27mins]
Case study
In a herd of Holstein cattle, a number of calves were showing symptoms of recurrent bacterial infections (e.g., pneumonia, enteritis, periodontitis), delayed wound healing, stunted growth and marked neutrophilia (elevated neutrophil count in blood tests).
Following observation of chronic infections and poor response to standard treatments, genetic testing was performed. DNA analysis revealed a single point mutation in the CD18 gene, where adenine is replaced by guanine at nucleotide position 383. This genetic alteration leads to an amino acid substitution in the CD18 adhesion molecule, specifically changing aspartic acid to glycine at position 128 (D128G). Functional assays further demonstrated that neutrophils from affected animals exhibit impaired expression and activity of β2 integrins (CD11a, CD11b, CD11c/CD18), which are essential for leukocyte adhesion and migration. This dysfunction compromises the immune system’s ability to respond effectively to infections.
The diagnosis is Bovine Leukocyte Adhesion Deficiency (BLAD) — a congenital autosomal recessive disorder affecting immune cell function.
BLAD is caused by a point mutation in the CD18 gene, impairing the expression of β2 integrins on neutrophils. This defect prevents neutrophils from adhering to blood vessel walls and migrating to infection sites, leading to ineffective immune responses despite elevated neutrophil counts.
There is no cure for BLAD. Management focuses on supportive care, antibiotics to control infections, wound care and hygiene to prevent secondary infections, nutritional support to promote growth and immune function. However, due to the progressive and fatal nature of the disease, affected calves have a poor prognosis.
The focus is therefore on preventative management. Genetic testing of breeding stock to identify carriers of the BLAD mutation and selective Breeding. Eradication programs have been implemented in many countries to reduce the incidence of BLAD in Holstein populations.
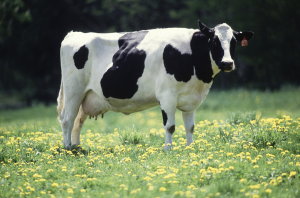